Introduction
Dental composites, or resin-based composites,is a cement made of synthetic resins1. It is a mixture of plastic (acrylic) resin that’s reinforced with a powdered glass filler
synonyms
- dental composite
- dental composite resin
- dental resin composite
- dental polymer composite
Evolution of composite
In the 1970s, the first commercially successful restorative composite resins (Concise [3M] and Adaptic [Dentsply Sirona]) were introduced. They were considered macrofills, containing very large filler particles and measuring zero to 5 μm. The irregular-edged, large particle size made them very difficult to polish even though they were rather strong.
In the 1980s, microfill composites made their debut, including Durafill VS (Kulzer) and Renamel (Cosmedent).3 They continue to have properties that are virtually the opposite of the macrofills: excellent polishability and aesthetics. However, most did not exhibit the strength required for use in occlusal load-bearing areas, with one notable exception: a microfill formulated for posterior teeth called Heliomolar (and later Heliomolar HB) (Ivoclar Vivadent).
During the 1990s, hybrids and the layering of composites became popular. The earliest hybrids were not easily polishable.4 Subsequent products (such as the original Esthet-X [Dentsply Sirona]) were stronger, but their aesthetics did not match today’s composite resin options.
The 2000s brought multiple new formulations along with better aesthetics. Translucent and opaque shades have allowed us to truly mimic tooth structure for the first time. In fact, many of today’s products create a need to buy kits that contain upwards of 30 shades. This has created problems for offices that needed to stock all of these shades; namely, that many of the shades would not be used during the product life span. In addition, as this millennium arrived, nanofilled composites were introduced. These consisted of nano particles (5 to 75 nm) and nanocluster fillers. They include particles from 5 to 20 nm in size.4,5 A smoother finish and shine resulted as well as stronger restorations.6 These products include Tetric EvoCeram (Ivoclar Vivadent) and Filtek Supreme Plus (3M).
Bulk-fill composites have become accepted by a larger population of dentists in the 2010s. Companies have promoted less polymerization shrinkage and a higher depth of cure up to 4.0 mm.7 The first highly accepted flowable bulk fill, SureFil SDR flow (Dentsply Sirona), was limited to use as a base under restorations.7 Newer flowable bulk-fill agents, including Tetric EvoCeram Bulk Fill (Ivoclar Vivadent) and Estelite Bulk Fill Flow (Tokuyama Dental America), require no capping layer of another composite. They show higher strength and aesthetics; however, some bulk fills can be more translucent. This can be a positive or negative attribute, depending on the situation.

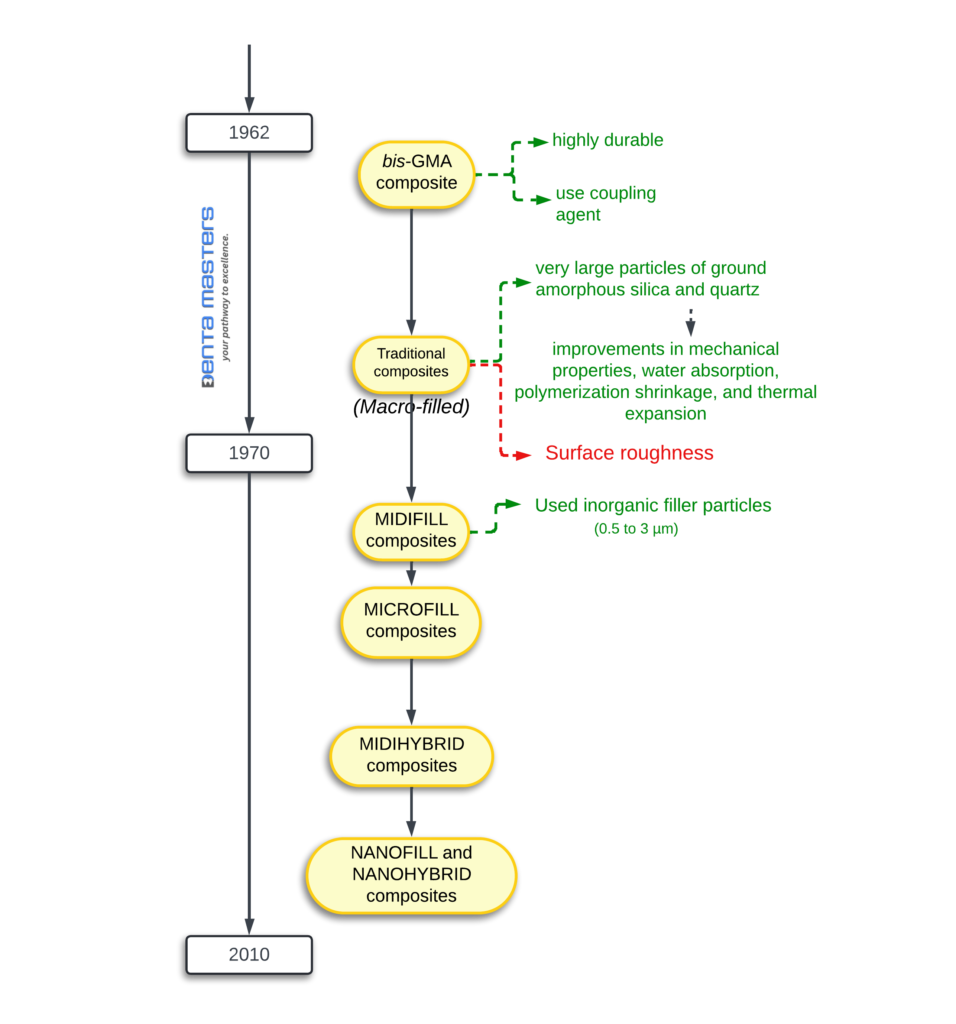
Revolutionary Change
This year, 2019,Tokuyama Universal Bond (Tokuyama Dental America) (Figure 4), is a truly universal agent that bonds to virtually everything. This includes direct, indirect, light-cure, self-cure, and dual-cure materials in self-etch, total-etch, and selective-etch modes. What makes this material a paradigm shifter is that no light curing, primers, or activators are needed. The clinician mixes equal parts A and B, applies it to dentin and enamel, and air dries it to polymerize the bonding agent prior to adding the composite of choice. Many dentists have not used a bonding agent without light curing since the early days of Tenure (DenMat) about 20 years ago.
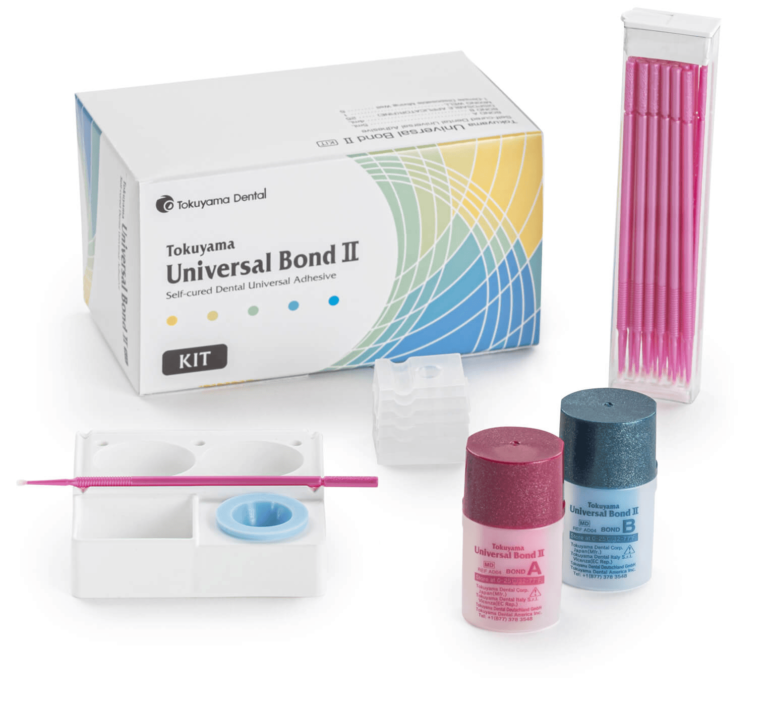
Tokuyama Universal Bond (Tokuyama Dental America) is a
next-generation bonding agent that bonds to all tooth surfaces and dental materials and does not require light activation.
Also, 2019 brings the introduction of another truly groundbreaking material, Omnichroma (Tokuyama Dental America). This is the first omnichromatic composite resin-based material that will match any tooth, any shade, on any patient. In an era when many composite materials require having multiple shades available for use in any given clinical situation, Omnichroma poses a unique opportunity for dental offices that cannot stock dozens of product shades for financial or space reasons. It is ideal for any office that values a quick, easy system that creates attractive, functionally sound restorations.
Omnichroma does not attempt to match the value of the tooth shade the way other composites do by using pigments and dyes. Instead, Tokuyama Dental America debuts its Smart Chromatic Technology with this product. The material uses uniformly sized spherical filler particles, as do the other composites in the Tokuyama Dental product line; however, Omnichroma fillers change the way that light is transmitted. Ambient light is altered along the red-to-yellow area of the color spectrum, allowing it to match the color of the patient’s surrounding dentition. It includes UDMA/TEGDMA monomers and 260-nm silica and zirconia fillers.
Omnichroma material characteristics include high polishability, excellent handling, and resistance to ambient light effects, highlights of other Tokuyama Dental America’s Estelite products. Wear and abrasion properties, including wear of the composite and opposing tooth structure, are also excellent.
The Omnichroma paste is opaque-white prior to curing, making the material more visible for the clinician during manipulation. The application of a light source during curing causes the material to blend with the color of the surrounding tooth. A chamfered margin is suggested to make marginal borders disappear. A single shade is all that is needed to match most posterior and anterior teeth. A blocking agent (Omnichroma Blocker [Tokuyama Dental America]) is available for extensive Class III and Class IV restorations. The function of the blocker is to reduce shade-matching interference caused by other objects in the background of the mouth, including the tongue, gingiva, and cheek.
Classification of composite
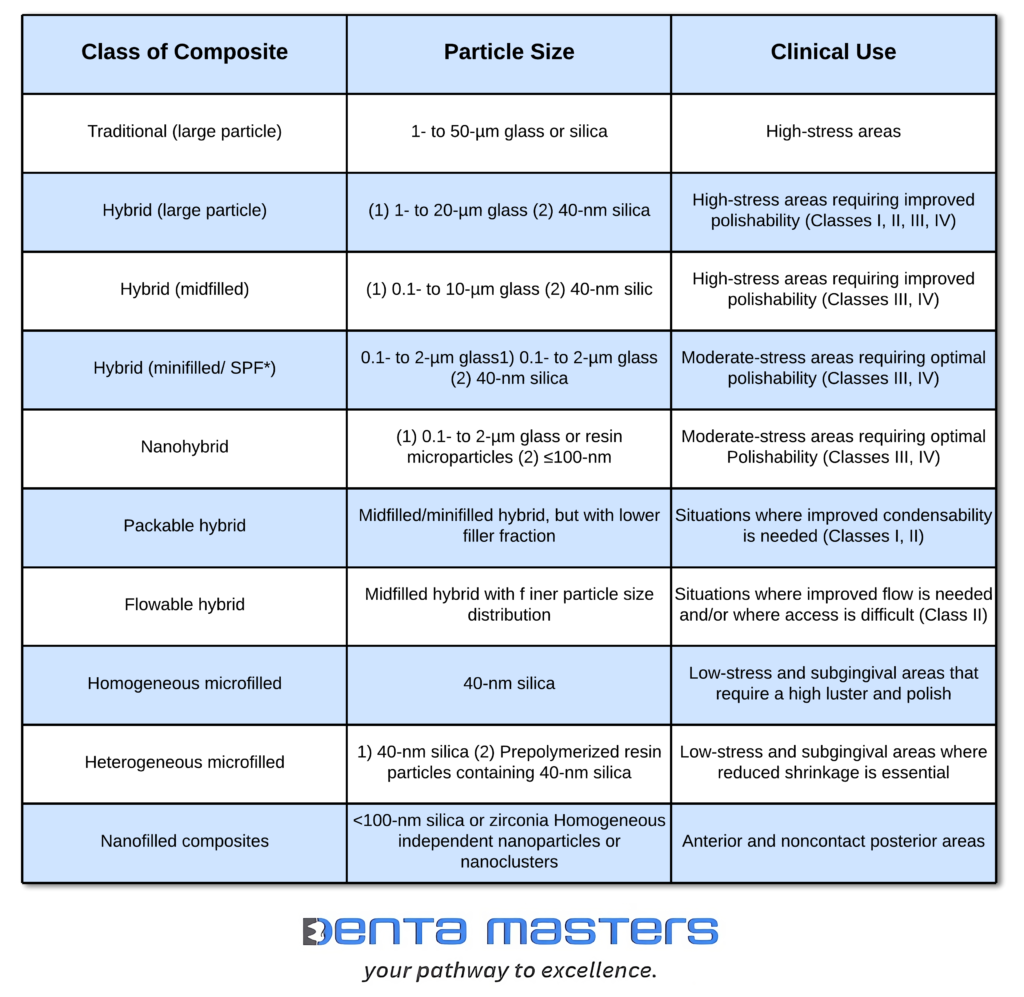
COMPOSITION
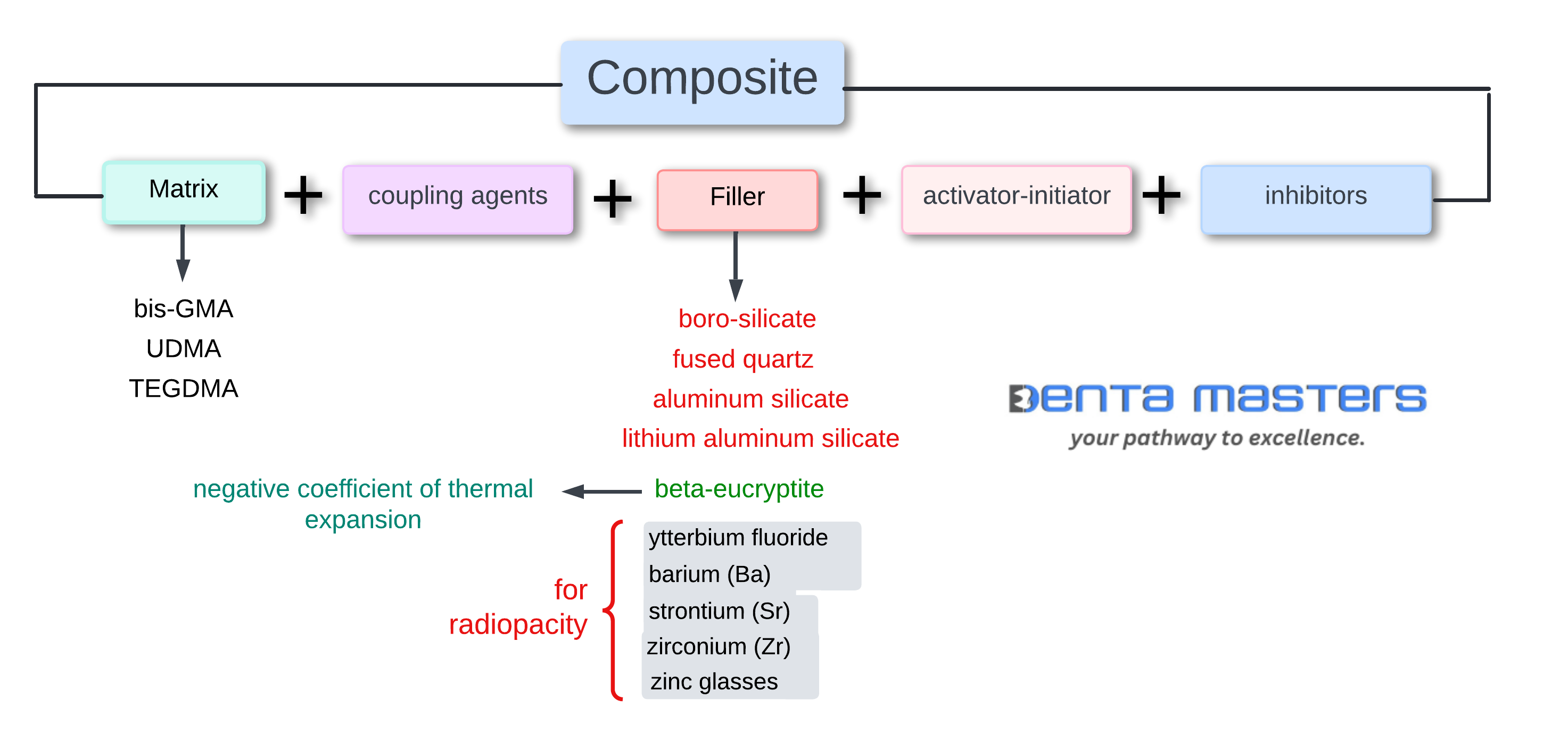
Matrix (mainly Polymer) | the bulk we need for restoration of a tooth surface |
filler | it increase the durablility of the material |
coupling agent | it helps in bonding of different material to each other |
activator or initiator | it starts the reaction that is required to convert the resin paste from a soft, mold able filling material to a hard, durable restoration. |
inhibititor | extend storage life and provide increased working time for chemically activated resins. |
Dental composites are made up of three major components:
resin matrix – a highly cross-linked polymeric
filler particles – glass, silica, crystalline, metal oxide
coupling agents – silane- to bond matrix and filler particles
In addition, dental composites contain –
activator-initiator system that is required to convert the resin paste from a soft, mold able filling material to a hard, durable restoration.
Pigments help to match the color of tooth structure. Ultraviolet (UV) absorbers and other additives improve color stability,
- inhibitors – extend storage life and provide increased working time for chemically activated resins by stoping polymerization.
MATRIX -
is based on dimethacrylate monomers (a blend of aromatic and/or aliphatic) such as
The “Bowen” monomer – bis-GMA (bisphenol A and glycidyl methacrylate). it is hydrophobic in nature
urethane dimeth acrylate (UDMA to form highly cross-linked, strong, rigid, and durable polymer structures). UDMA has a lower molecular weight (M = 470 g/mol) and is more flexible than BisGMA.
UDMA and bis-GMA are highly viscous and are difficult to blend and manipulate.
Thus, it is necessary to use varying proportions of lower-molecular-weight highly fluid monomers such as triethylene glycol dimethacrylate – TEGDMA
For example, a blend of 75% bis-GMA and 25% TEGDMA by weight has a viscosity of 4300 centipoise, whereas the viscosity of a 50% bis-GMA/50% TEGDMA blend is 200 centipoise (similar to thin syrup).
Unfortunately, these smaller, diluent monomers undergo greater polymer ization shrinkage, and the greater the risk of eventual leakage in marginal gaps. partially offsetting the advantage of using large monomers such as bis-GMA.
bis-GMA and TEGDMA, the refractive indices are approximately 1.55 and 1.46.
mixture of the two components in equal proportions by weight yields a refractive index of approximately 1.50.
Most of the glasses and quartz used for fillers have refractive indices of approxi mately 1.50, which is adequate for sufficient translucency.
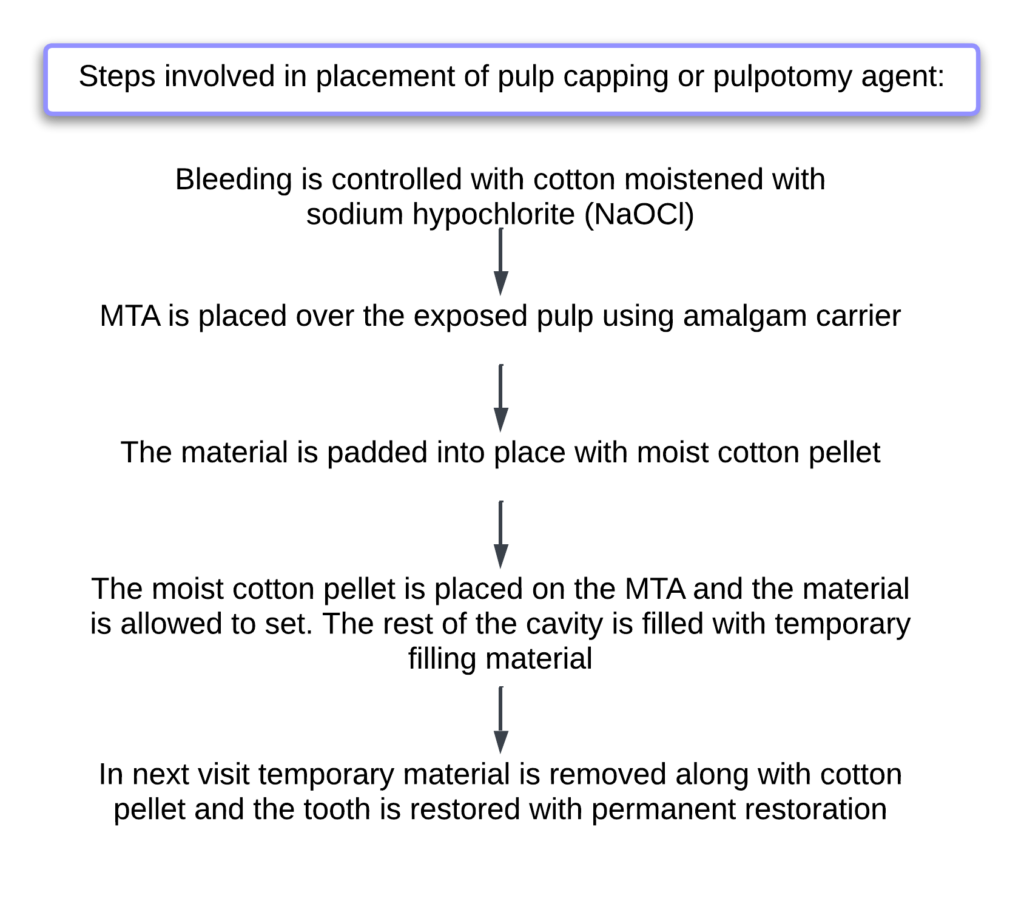
Filler
fillers are employed to strengthen and reinforce composites as well as to reduce curing shrink age and thermal expansion (generally between 30% to 70% by volume or 50% to 85% by weight of a composite).
“soft glass” and borosilicate “hard glass”, fused quartz, aluminum silicate, lithium aluminum silicate (beta-eucryptite, which has a negative coefficient of thermal expansion).
ytterbium fluoride, and barium (Ba), strontium (Sr), zirconium (Zr), and zinc glasses. The latter five types of f illers impart radiopacity because of their heavy metal atoms.
Quartz has the advantage of being chemically inert but it is also very hard, making it abrasive to opposing teeth or restorations as well as difficult to grind into very fine particles; thus, it is also difficult to polish.
Function of Fillers
Reinforcement –
increases physical and mechanical properties that determine clinical performance and durability, such as –
compressive strength.
tensile strength.
modulus of elasticity (i.e., stiffness or rigidity).
toughness.
As the volume fraction of filler approaches approximately 70%, abrasion and fracture resistance are raised to levels approaching those of tooth tissue,
Reduction of polymerization shrinkage/contraction.- Increased filler reduces curing shrinkage in proportion to filler volume fraction.
Reduction in thermal expansion and contraction. – Increased filler decreases the overall coefficient of thermal expansion of the composite because glass and ceramic fillers thermally expand and contract less than do polymers. As the overall expansion coefficient decreases with filler loading and approaches that of tooth tissue, less interfacial stress is produced because of differential volumetric changes while an individual consumes hot and cold foods and beverages.
Control of workability/viscosity. – Fluid liquid monomer + filler → a paste. The more filler, the thicker is the paste. Filler loading, filler size, and the range of particle sizes and shapes all affect mark edly the consistency of a composite paste, which in turn strongly affects clinical manipulation and handling properties, such as ease of mixing and sculpting, tackiness, and the tendency to either hold a shape or to minimize slumping.
Decreased water sorption. Increased filler loading decreases water sorption. Absorbed water softens the resin and makes it more prone to abrasive wear and staining.
Imparting radiopacity. Resins are inherently radiolu cent. However, leaking margins, secondary caries, poor proximal contacts, wear of proximal surfaces, and other problems cannot be detected unless ade quate radiographic contrast can be achieved. Thus, radiopacity is an important property. Radiopacity is most often imparted by adding certain glass filler par ticles containing heavy metal atoms.
Although glass fillers containing metals of a high atomic number provide radiopacity, they are not as inert as quartz and amorphous silica and are slowly leached and weakened in acidic liquids such as citrus juices, high pH solutions, and other oral fluids. The glass filler is also attacked over time by caries-protective acidulated phosphate fluoride solutions or gels.However, the implication is that glass filled composites will gradually become more susceptible to abrasive wear and hence they will have a shorter functional lifetime compared with silica-reinforced resins.
small filler advantge -
If particle size is uniform, no matter how tightly packed the particles are, spaces will exist among them, as illustrated by the example of the void spaces in a box filled with spheres of the same size. However, if smaller particles are inserted among the larger spheres(bis-gma, udma,tegdma), the void space can be reduced.
Another advantage of using small particles is that they improve esthetics (appearance) and smoothness to the tongue (polishability).
Particles larger than the wavelength of visible light cause light scattering.Scattering increases opacity and produces a visibly rough texture when the particles are exposed at the surface. A roughened surface also tends to accumulate stains and plaque
Classifications of Filled Resins
Macrofilled Composites
The first type of dental composite to be developed in the 1960s
The filler is a quartz material with particle sizes of 10 to 25 μm.
Filler content is 70% to 80% by weight.
Because the filler phase is much denser than the resin phase, the volume percentage is typically 10% to 15% lower than the weight percentage.
The large size of the filler particles in macrofilled composites results in a restoration that feels rough to the dental explorer and can appear rough to the eye.
The likelihood of plaque accumulation and staining is greater
composite will turn slightly gray when rubbed with an instrument.
Macrofills were used before dentinal bonding systems were developed; placing them in posterior teeth resulted in postoperative sensitivity, leakage, and recurrent decay
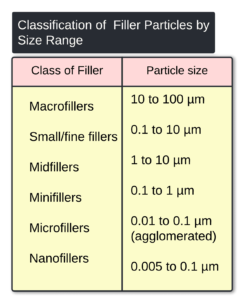
Small (Fine) Particle Composites.
Small-particle composites have mean particle diameters between 0.1 and 10 µm (mini f iller and midifiller).
These composites, while more polishable than traditional macrofilled composites (i.e., 10 to 100 µm), cannot be polished to a high gloss.
However, filler loadings are as high as or higher (77% to 88%) than those of macro filled composites, which provides a high degree of hardness and strength but also brittleness.
Its excellent balance among polishability, appearance, and durability make this category suitable for general anterior use.
Microfilled Composites
These composites are recommended for low stress bearing class 3 and class 5 restorations, in which a high polish and esthetics are most important.
microfilled composites exhibit more water sorption and thermal expansion than microhybrid compos ites or nanocomposites.
Microfilled composites are agglom erates of 0.01- to 0.1-µm inorganic colloidal silica particles embedded in 5- to 50-µm resin filler particles.
The problems of surface roughening and low translucency associated with traditional and small-particle composites can be overcome through colloidal silica particles
compound such as SiCl4 is burned in an oxygen/hydrogen atmosphere to form macromolecular chains of colloidal silica resulting in amorphous silica (colloidal, noncrystalline SiO2)
which produces highly polishable esthetic composite restorations. because of their extremely small size, have extremely large surface areas so have high viscocity and make clinical manipulation difficult.
a microfilled com posite, will not shrink as much as expected based on the total resin volume. a major shortcoming is that the bond between the composite particles and the clinically cured matrix is relatively weak, facilitating wear by a chipping mechanism.
Because of this deficiency, microfilled composites are not gen erally suitable for use as stress-bearing surfaces.
This is to be expected, because 40% to 80% by volume of the restorative material is made up of resin, resulting in greater water sorption, a higher coefficient of thermal expansion, and decreased elastic modulus.
In addition, the weak bond of the prepolymerized particles to the clinically cured resin matrix results in decreased tensile strength
In addition, in the longer term, if microfilled composites are placed in wear-prone areas, they eventually break down and wear at a rate too fast for accept able clinical performance.
Hybrid Composites
formulated with mixed filler systems containing both microfine (0.01 to 0.1 µm) and fine (0.1 to 10 µm) particle f illers in an effort to obtain even better surface
Thus, they are also suitable for restoring certain high-stress-sites where esthetic considerations dominate—for example, incisal edges and small non-contact occlusal cavities. They are widely used for anterior restorations, including class IV sites
The smaller microfiller sizes increase the surface area, which generally increases the viscosity and requires a decrease in overall filler loading as compared with small particle composites. A polished surface is shown in Figure
Nanofilled Composite
More recently, nanoparticles (1 to 100 nm) have been fabricated by a different method from the pyrolytic precipitation process used for colloidal silica.
This allow primary particles to be surface-coated (with γ methacryloxypropyltrimethoxysilane, for example) prior to becoming incorporated into three-dimensional macromolecule chains, thereby preventing or limiting particle agglom eration into large networks and driving up viscosity.
In essence, the particle size is similar to that in microfilled com posites, but the difference is that the particles in microfilled composites are in three-dimensional agglomerates or net works that increase viscosity whereas those in nanofilled composites are mostly discrete and have a minimal effect on viscosity.
these composites have optical properties and superior polishability like those of microfilled composites,
Classification of Composites by Manipulation
Flowable Composites -
A modification of the small-particle composite and hybrid composite results in the so-called flow able composites,
T hese resins typically have a lower viscosity through a reduced f iller loading, which enables the resin to flow readily, spread uniformly, intimately adapt to a cavity form, and produce the desired dental anatomy
This improves the clinician’s ability to form a well-adapted cavity base or liner, especially in class II posterior preparations and other situations in which access is difficult.
they are inherently inferior in mechanical properties owing to the lower filler loading and higher susceptibility to wear and other forms of attrition.
The properties and clinical uses of flowable composite materials are similar to those of the so-called compomers, which are hybrids between resin composites and glass ionomer materials
Condensable (Packable) Composites
Because of their highly plastic, pastelike consistency in the precured state, compos ites cannot be packed vertically into a cavity into a cavity in such a way that the material flows laterally as well as vertically to ensure intimate contact with the cavity walls.
Thus, condensable composites (also known as packable composites) were devel oped by adjusting their filler distribution to increase the strength and stiffness of the uncured material and provide a consistency and handling characteristics similar to those of lathe-cut amalgam.
/condensable characteristics are derived from the inclusion of elongated, fibrous filler particles of about 100 µm in length and/or rough-textured surfaces or branched geometries that tend to interlock and resist flow.
Coupling Agent
it helps filler particles be bonded to the resin matrix.
this is a difunctional surface-active compound that adheres to filler particle surfaces and also coreacts with the monomer forming the resin matrix.
the most com mon of which are organic silicon compounds called silane coupling agents.
During the filler treatment process, the methoxy groups hydrolyze to generate hydroxyl groups through an acid-or base-catalyzed reaction.
It forms an interfacial bridge that strongly binds the filler to the resin matrix.
It enhances the mechanical properties of the composite and minimizes the plucking of the
Initiators and Accelerators
The curing of composites is triggered by light.
Light activation is accomplished with blue light at a peak wavelength of about 465 nm, which is absorbed usually by a photosensitizer, such as camphorquinone, added to the monomer mixture during the manufacturing process in amounts varying from 0.1% to 1.0%.
In methacrylate composites, free radicals are generated upon activation.
The reaction is acceler ated by the presence of an organic amine.
The amine and the camphorquinone are stable in the pres ence of the oligomer at room temperature, as long as the composite is not exposed to light.
Chemical activation is accomplished at room tem perature by an organic amine (catalyst paste) reacting with an organic peroxide (universal paste) to produce free radicals, which in turn attack the carbon double bonds, causing polymerization. Once the two pastes are mixed, the polymerization reaction proceeds rapidly.
Activation/initiation System
Both monomethacrylate and dimethacrylate monomers polymerize by the addition polymerization mechanism initi ated by free radicals,
Free radicals can be generated by
chemical activation
external energy activation (heat, light, or micro wave).
Chemically Activated (Self-Cure) Resins
supplied as two pastes –
one of which contains the benzoyl peroxide initiator
an aromatic tertiary amine activator
When the two pastes are mixed together, the amine reacts with the benzoyl peroxide to form free radicals, and addition polymerization is initiated.
problem with chemical activation –
during mixing it is almost impossible to avoid incorporating air into the mix, thereby forming pores that weaken the structure and trap oxygen, the formation of an oxygen inhibited layer occur. which inhibits polymerization during curing. During the initial stage of a polymerization process, the polymerization is inhibited by oxygen because the reactivity of oxygen to a radical is much higher than that of a monomer. During the inhibition period, this oxygen diffuses into the liquid resin and is consumed by the radicals formed.
operator has no control over the working time after the two components have been mixed.
Photochemically Activated (Light-Cure) Resins
supplied as a single paste con tained in a lightproof syringe.
The free radical initiating system, consisting of a photosensitizer and an amine initiator, is contained in this paste.
As long as these two components are not exposed to light, they do not interact.
expo sure to light in the blue region (wavelength of about 468 nm) produces an excited state of the photosensitizer, which then interacts with the amine to form free radicals that initiate addition polymerization
Camphorquinone (CQ) is a commonly used photosensitizer that absorbs blue light with wavelengths between 400 and 500 nm.
amine initiators are suitable for interac tion with CQ, such as dimethylaminoethyl methacrylate (DMAEMA),
In addition to avoiding the porosity of chemically activated resins, light-cured materials allow the operator to complete insertion and contouring before curing is initiated.
Further more, once curing is initiated, an exposure time of 40 seconds or less is required to light-cure a 2-mm-thick layer, as compared with several minutes for chemically-cured materi als.
limitations –
First, they must be placed incrementally when the bulk exceeds approximately 2 to 3 mm because of the limited depth of light penetration.
cost of the light-curing unit, the need for eye protection,
Dual-Cured Resins
to overcome limits on curing depth and problems associated with light curing is to combine chemical curing and visible-light curing components in the same resin.
consist of two light-curable pastes –
one containing benzoyl peroxide
an aromatic tertiary amine accelerator
they set very slowly when mixed via the self-cure mechanism. The cure is then acceler ated on “command” via light-curing promoted by the amine/ CQ combination.
The major advantage of this system is assur ance of completion of cure throughout, even if photocure is inadequate.
major disadvantage is porosity caused by the required mixing.
less color stability than with the photocure resins owing to the aromatic amine accel erators, but this is still better than for self-cure systems, since the concentration of the accelerators is reduced compared with self-cure resins.
Depth of Cure and Exposure Time.
light absorption and scattering in resin composites reduces the power density and degree of conversion (DC) exponentially with depth of penetration.
Intensity can be reduced by a factor of 10 to 100 in a 2-mm-thick layer of composite.
This reduces monomer con version to an unacceptable level at depths greater than 2 to 3 mm. the surface must be irradiated for a longer time to deliver sufficient power density well below the surface.
Light attenuation can also vary considerably from one type of composite to another, for example, on the opacity, filler size, filler concentration, and pigment shade.
darker shades and/or more opaque resins require longer curing times.
for proximal boxes – causing incomplete curing, due to absorption and scattering of Light as it passes through tooth structure, especially dentin. when one attempts to polymerize the resin through tooth structure, the exposure time should be increased by a factor of 2 to 3 to compensate for the reduction in light intensity.
Inhibitor
added to minimize or prevent spontaneous or accidental polymerization of monomers.
Inhibitors have a strong reactivity potential with free radicals.
If a free radical is formed, for example, by brief exposure to room lighting when the material is dispensed, the inhibitor reacts with the free radical faster than the free radical can react with the monomer. This prevents chain propagation by terminating the reaction before the free radical is able to initi ate polymerization.
After all of the inhibitor is consumed, chain propagation can begin.
A typical inhibitor is butylated hydroxytoluene (BHT). BHT and similar free radical scavengers are also used as food preservatives to prevent oxi dation and rancidity.
Thus, inhibitors have two functions: to extend the resin’s storage life and to ensure sufficient working time.
Curing Shrinkage and Shrinkage Stress
Curing shrinkage arises as the monomer is converted to polymer and the space it occupies reduces.
T he polymerization shrinkage and resultant stress can be affected by the
total volume of the composite material,
type of composite,
polymerization speed, and
ratio of bonded/nonbonded surfaces or the configuration of the tooth preparation (C-factor).
These stresses weakening the bond, and eventu ally producing a gap at the restoration margins.
the risk for marginal leakage and the ensuing problems of marginal staining and secondary caries are exacerbated.
greatest problems of compos ites used for class II and class V restorations.
the problem is solved by 2 methods –
larger monomers used to “dilute” the number of double bonds that need to be reacted
addition of inorganic fillers that do not enter into the polymerization process, although they do bond to the polymer.
Unreacted C═C Bonds
Not only can composite materials be placed in layers and bonded together but also new composite will bond to old composite. Why?
Not all C═C bonds react when a dental composite sets.
Typically, only about 50% of the C═C bonds react.
Therefore, it is possible to repair or add to a composite restoration by cleaning the surface and then properly adding new material.
Some of the unreacted C═C bonds in the old material will react with the setting matrix of the new material.
The strength of the bond is much lower than that of a newly placed, solid piece of material, but adding new composite material to an old composite is not uncommon.
Reduction of Shrinkage Stresses
light-activated resins have overcome many of the deficiencies of chemically activated resins, including –
lack of control over working time
color shift (yel lowing),
porosity from mixing the two-part system.
the internal pores in chemically cured resins act to relax residual stresses that build up during curing (the pores enlarge during hardening and reduce the concentration of stresses at the margins).
the slower curing rate of chemi cal activation allows a larger portion of the shrinkage to be compensated by internal flow among the developing polymer chains before extensive cross-linking occurs (i.e., before gela tion).
After the gel point, stresses cannot be relieved but instead continue to increase and concentrate within the resin and the tooth structure adjacent to the bonded surfaces.
Two general approaches to overcome the problem of stress concentration and marginal failure experienced with light-activated resins:
reduction in volume contraction by altering the chemistry and/or com position of the resin system, and
clinical techniques designed to offset the effects of polymerization shrinkage.
Incremental Buildup and Cavity Configuration
During curing, composite leaves the bonded cavity surfaces in a state of stress;
the nonbonded, free surfaces of composte contracting inward toward the bulk of the material to relax some of the stress.
A layering technique in which the restoration is built up in increments, curing one layer at a time, effectively reduces polymerization stress by minimiz ing the C-factor.
thinner layers reduce bonded surface area and maximize nonbonded surface area, thus minimizing the associated C-factor.
limitations on the depth of cure of photoinitiated resins dictate the use of incremental buildup of the composite.
Thus, an incremental technique overcomes both limited depth of cure and residual stress concentration but adds to the time and difficulty of placing a restoration
Soft-Start
providing an initial low rate of polymerization, thereby extending the time available for stress relaxation before reaching the gel point.
using a soft-start technique, whereby curing begins at low light intensity and finishes with high intensity.
This allow slow initial rate of polymerization and a high initial level of stress relaxation during the early stages and it ends at the maximum intensity once the gel point has been reached.
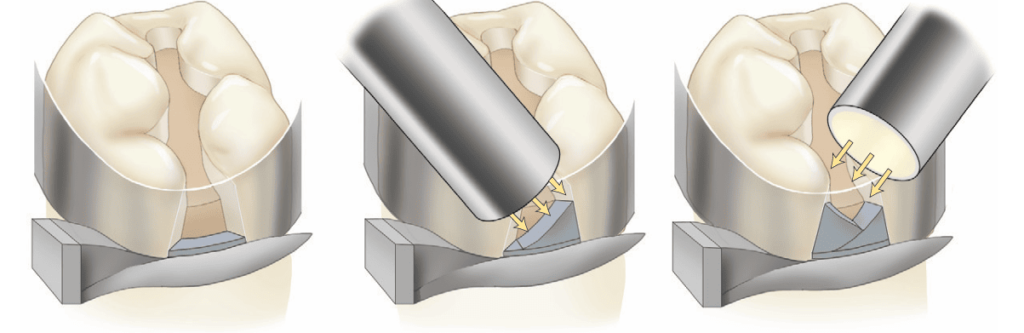
Ramped Curing
ramping employs stepwise, linear, or exponential modes.
In delayed curing, the restoration is initially incompletely cured at low intensity.
The clinician then sculpts and contours the resin to the correct occlusion and later applies a second exposure of light for the f inal cure. This delay allows substantial stress relaxation to take place.
The longer the time available for relaxation, the lower is the residual stress.
Biocompatibility of Composites
biocompatibility usually relate to the effects on the pulp from two aspects:
- the inherent chemical toxicity of the material
A chemical insult to the pulp from composites is possible if components leach out or diffuse from the material and subsequently reach the pulp.
Inadequately cured composite materials at the floor of a cavity can serve as a reservoir of diffusible components that can induce long-term pulp inflam mation. This situation is of particular concern for light activated materials.
If a clinician attempts to polymerize too thick a layer of resin or if the exposure time to the light is inadequate (as discussed previously), the uncured or poorly cured material can release leachable constituents adjacent to the pulp.
Nonetheless, adequately polymerized composites are relatively biocompatible because they exhibit minimal solubility, and unreacted species are leached in very small quantities. From a toxicological point of view, these amounts should be too small to cause toxic reactions.
the marginal leakage of oral fluids.
marginal leakage might allow bacterial ingrowth
these microorganisms may cause secondary caries or pulp reactions.
Bisphenol A Toxicity
has been shown to be a xenoestrogen, a synthetic compound that mimics the effects of estrogen by having an affinity for estrogen receptors.
BPA and other endocrine-disrupting chemicals (EDCs) have been shown to cause reproductive anomalies, especially in the developmental stages of fetal wildlife.